Background
Whether migratory birds will respond successfully to rapid climate change is unclear. Birds migrate to take advantage of seasonal peaks in resource availability: Food and habitat become abundant quickly during summer at northern latitudes, but decline quickly in the fall. Timely arrival on the summer range is vital for many species (Alerstam and Hedenström 1998).
While time is limiting during migration, energy often is as well. Birds can stop periodically to forage in suitable habitats–a behavior commonly referred to as ‘stopover’–allowing them to replenish energy stores when necessary. Some birds, such as soaring migrants, can use wind and uplift to directly subsidize the energetic costs of flight during migration (Katzner et al. 2015). The energy landscape, a concept formalized relatively recently, can help explain the way animals move (Shepard et al. 2013). Like water flowing downhill, animals will tend to follow the path of least resistance; that is, animals travel through areas that tend to minimize energy expenditure. The environment is dynamic, as are the ways in which animals respond to it, hence the prevalence of long-distance migration.
While the energy expended during migration is an important factor for birds’ migration strategies, the energy stores acquired before departure are of similar importance. This is, in part, because many birds are pushed to their physiological limits to make several thousand kilometer trips twice annually. Not surprisingly, the availability of food resources often explains where birds overwinter.
As climate change progresses, we will likely see earlier springs and northwards shifts of southern habitats. Earlier springs mean that peaks of prey availability at northern latitudes will occur earlier, potentially becoming desynchronized with the arrival of dependent migrants–formally known as phenological disjunction (Crick 2004). If birds are already pushed to their limits to successfully migrate, they may not be able to deposit sufficient energy stores in even more limited time frames. Of course, this raises the question of whether birds will be able to respond in a timely manner, maintaining suitable alignment between arrival on the breeding grounds and peaks in resource availability.
There has long been evidence of the genetic programming of bird migration (Biebach 1983), but it is not clear how flexible birds are in shifting behaviors, such as departure dates. For example, if evolution by natural selection is the only mechanism for arriving on the breeding grounds earlier, bird populations may not be able to respond at pace with climate change because responses would not occur for several generations.
An alternative mechanism would be individuals adapting their behaviors. The cognitive and learning capabilities of animals have been studied for some time, and birds have been shown to improve their migratory performance within a lifetime (Sergio et al. 2014). Improved migratory performance results from energy and/or time minimization. Of all the possible strategies for minimizing time and energy expenditure, perhaps the simplest is just not migrating as far. Switching to a more northern overwintering area is called ‘shortstopping’–a quite intuitive name, given that it simply requires a bird to stop early in its southward migration. This seems to be exactly what some whooping cranes (Grus americana) have begun to do in response to climate change.

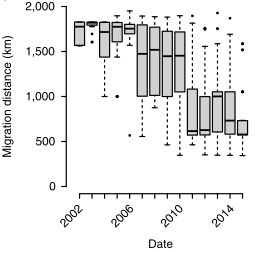
Whooping cranes were recently reintroduced into the US and are unique in that many were trained to migrate between summering and overwintering areas, like Wisconsin and Florida, being led by ultralight aircraft. Some cranes were trained to three distinct overwintering areas, but have since used 65 areas that they established independent of the training (Figure 1; Teitelbaum et al. 2016). Note that the appearance of shortstopping in these cranes was incredibly abrupt (Figure 2; Teitelbaum et al. 2016).
Question
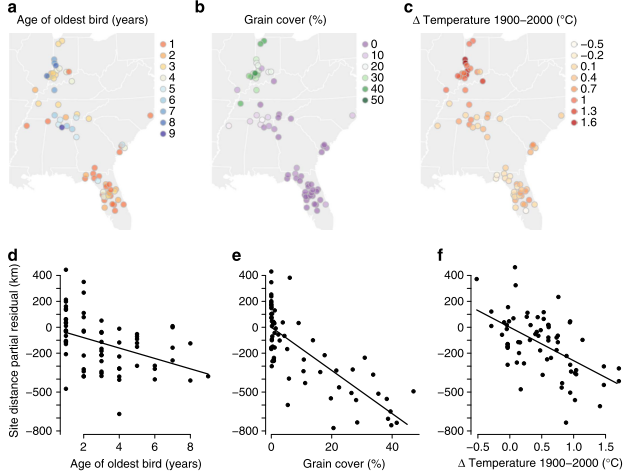
Teitelbaum et al. (2016) set out to determine the mechanisms for the abrupt appearance of shortstopping in a reintroduced population of whooping cranes. Teitelbaum et al. (2016) tested the effects of age, human land use, and temperature as mechanisms for the emergence of shortstopping in whooping cranes (Figure 3). These factors reflect the ability of cranes to learn from experience and by social interaction, as well as general food and thermoregulatory limitations.
Evidence

The authors found that older whooping cranes have a higher probability of shortstopping, but younger birds learn it over time (Figure 4). This shows that innovation can arise with age in birds, which is consistent with findings based on primate behavior (Reader and Laland 2001). Other species organize in groups with complex social structure led by older individuals. The older individuals’ experience can provide an adaptive advantage to all members of the group (McComb et al. 2001).
While it is interesting that older birds shortstop and the younger birds learn from that behavior, no birds should shortstop unless there is suitable overwintering habitat closer to the breeding grounds than previous overwintering areas. While Teitelbaum et al. (2016) found that warming at northern sites of less than one degree Celsius more than warming at southern sites did influence shortstopping, one degree is hardly enough to make winter temperatures of the Midwest more hospitable for cranes. More likely, older cranes saw it suitable to stop sooner because of human land use patterns. Greater percent grain coverage at northern sites (Figure 2), likely coupled with warmer temperatures, drove the older cranes to shortstop (Teitelbaum et al. 2016).
Further questions
Now, many whooping cranes expend much less energy and time during migration. Assuming that outweighs the costs associated with overwintering further north, shortstopping may provide them with a fitness advantage. Understanding the fate of individuals’ offspring from a latitudinal gradient of overwintering areas could provide an understanding of potential differential fitness between northern and southern overwintering populations. Additionally, as migration has genetic controls, tracking genetic responses to this shortstopping behavior could reveal how a learned behavior can set a population on some new eco-evolutionary trajectory. Genotyping shortstoppers and non-shortstoppers at some of the key migration loci over time would be a potential method for observing any genetic responses to shortstopping.
Lastly, Teitelbaum et al. (2016) used VHF transmitters to relocate cranes. VHF technology is long obsolete in the realm of movement ecology. Deploying GPS transmitters, perhaps in tandem with triaxial accelerometers, could allow energy expenditure to be modeled throughout the year to determine exactly how overwintering behaviors could lead to certain fitness payoffs.
Further reading
Whooping cranes have greater cognitive abilities than many other birds, so they may have a greater capacity to make behavioral responses to climate change. For an example of a species, (Pied Flycatcher Ficedula hypoleuca) that is less flexible, see: Both, C., and M. E. Visser. 2001. Adjustment to climate change is constrained by arrival date in a long-distance migrant bird. Nature 411:296—298. doi:10.1038/35077063
Everything an animal does will have some effect on fitness. Linking animal movement directly to fitness is an area of research that I think will expand soon with improvements in tracking technology. Methods for investigating the link between animal movement and fitness with empirical data are lacking, but for a taste, see:
Lewis, M. A., and P. Moorcroft. 2001. ESS analysis of mechanistic models for territoriality: the value of scent marks in spatial resource partitioning. Journal of Theoretical Biology 210:449—461. Or their book Mechanistic Home Range Analysis (https://press.princeton.edu/titles/8338.html)
In case you’ve missed the movie Fly Away Home from the mid 90’s about teaching geese to migrate: https://www.youtube.com/watch?v=Ui9th4nhvoY
References
- Alerstam, T., and A. Hedenström. 1998. The development of bird migration theory. Journal of Avian Biology 29:343—369. doi:10.2307/3677155
- Biebach, H. 1983. Genetic Determination of Partial Migration in the European Robin (Erithacus rubecula). The Auk 100:601—606. https://www.jstor.org/stable/4086461
- Crick, H. Q. P. 2004. The impact of climate change on birds. Ibis 146:48—56. doi:10.1111/j.1474-919X.2004.00327.x
- Katzner, T. E., P. J. Turk, A. E. Duerr, T. A. Miller, M. J. Lanzone, J. L. Cooper, D. Brandes, J. A. Tremblay, and J. Lemaître. 2015. Use of multiple modes of flight subsidy by a soaring terrestrial bird, the golden eagle Aquila chrysaetos, when on migration. Journal of Royal Society Interface 12:20150530. doi:10.1098/rsif.2015.0530
- McComb, K., C. Moss, S. M. Durant, L. Baker, and S. Sayialel. 2001. Matriarchs act as repositories of social knowledge in African elephants. Science 292:491—494. doi:10.1126/science.1057895
- Moorcroft, D., D. Moorcroft, M. J. Whittingham, M. J. Whittingham, R. B. Bradbury, R. B. Bradbury, J. D. Wilson, and J. D. Wilson. 2002. The selection of stubble fields by wintering granivorous passerine birds reflects vegetation cover and food abundance. J. Appl. Ecol 39:535—547. doi:10.1046/j.1365-2664.2002.00730.x
- Reader, S., and K. Laland. 2001. Primate Innovation: Sex, Age and Social Rank Differences. International Journal of Primatology 22:787—805. doi:10.1023/A:1012069500899
- Sergio, F., A. Tanferna, R. De Stephanis, L. L. Jiménez, J. Blas, G. Tavecchia, D. Preatoni, and F. Hiraldo. 2014. Individual improvements and selective mortality shape lifelong migratory performance. Nature 515:410—413. doi:10.1038/nature13696
- Shepard, E. L. C., R. P. Wilson, W. G. Rees, E. Grundy, S. A. Lambertucci, and S. B. Vosper. 2013. Energy landscapes shape animal movement ecology. The American Naturalist 182:298—312. doi:10.1086/671257
- Teitelbaum, C. S., S. J. Converse, W. F. Fagan, K. Böhning-Gaese, R. B. O’Hara, A. E. Lacy, and T. Mueller. 2016. Experience drives innovation of new migration patterns of whooping cranes in response to global change. Nature Communications 7:12793. doi:10.1038/ncomms12793